Research
About: my Basic Philosophy
What DNA sequence reveals is no less than astounding! Not only can the sequence reveal the identity of microbial members present, but it can inform evolution, the relatedness of all things living and how ‘life’ got where it is today. While great, we also like to use this knowledge to help further the protection of the environment. Over the past ten years we have pursued this topic on three distinct environmental microbiological fronts.
The first began with an examination of a living stromatolite along the shore of a Yellowstone National Park hot spring. Stromatolites are laminated, accretionary structures found throughout the rock record of Earth’s history and are thought to be capable of forming biotically or abiotically. We have shown that Yellowstone stromatolites form by a ‘building’ cyanobacterium that makes a silica-based, laminated ‘rock’, subsequently ‘inhabited’ by a host of diverse, tenant microbiota. They are arguably the best examples on Earth of a living stromatolite–better than those found in the waters of the Bahamas and Shark Bay, Australia. From an environmental protection standpoint, it may be possible to cultivate this ‘builder’ cyanobacterium, add it to, for example, the crushed rock tailings of a mine-works, and subsequently convert what is a potentially hazardous mine tailings pile into a stromatolite–tailings locked up in a new kind of rock. This has the potential to minimize further metal leaching from spent mine tailings.
A second area of research began with the basic question, how does a metal, the steel of tanks and pipes, rot? For years this was thought to be chemical corrosion, but we now know that a lot of corrosion is actually microbially influenced corrosion (MIC). Microbes in a tank, or lining a pipe as a biofilm on the walls, can use trace amounts of water, a carbon source in the pipeline (oil, gas, or other hydrocarbon fuels), trace amounts of hydrogen and the metals in the steel alloys themselves as carbon and energy sources. In an active state of metabolism, the cells of MIC use these constituents to actively ‘rot’ the steel. Initially, a pit forms, which grows with time to cause pipe or tank failure. Understanding the microbial underpinnings of MIC can help to prevent both oil, gas and other hydrocarbon infrastructure from environmentally catastrophic failure. Another example is that the steel within a bridgeworks can fail, collapsing the bridge; both of these scenarios have recently occurred.
The third area of research involves the conversion of a traditional microbial wastestream into a beneficial revenue stream–a classic environmental engineering problem. Process wastewater from a brewery is ‘treated’ for excess carbon and/or nitrogen content by microbes in a wastewater treatment plant. Typically, the spent microbes are collected, dewatered, and wasted to a landfill. That wasted biomass can have greater than 60% protein content, which can be put to a more beneficial use as a source of protein to feed farmed fish in aquaculture. This ‘process biomass’ has the added benefit of not coming from domestic waters and thus contains very few pathogens; it is an ideal source of protein for aquaculture. We have characterized this biomass, found it to be a healthy feed alternative for aquaculture, and have converted an environmental waste into a beneficial use.
These three lines of research are all great for environmental protection in their own right. What is more important, is that the knowledge of the microbiota present in each environment from a DNA sequence perspective better informs us about the kinds of microbes that make up the ‘Tree of Life.’ Thus, we learn more about the processes of life, where life came from and where life may be possible elsewhere–on other planetary bodies of our solar system or the universe!
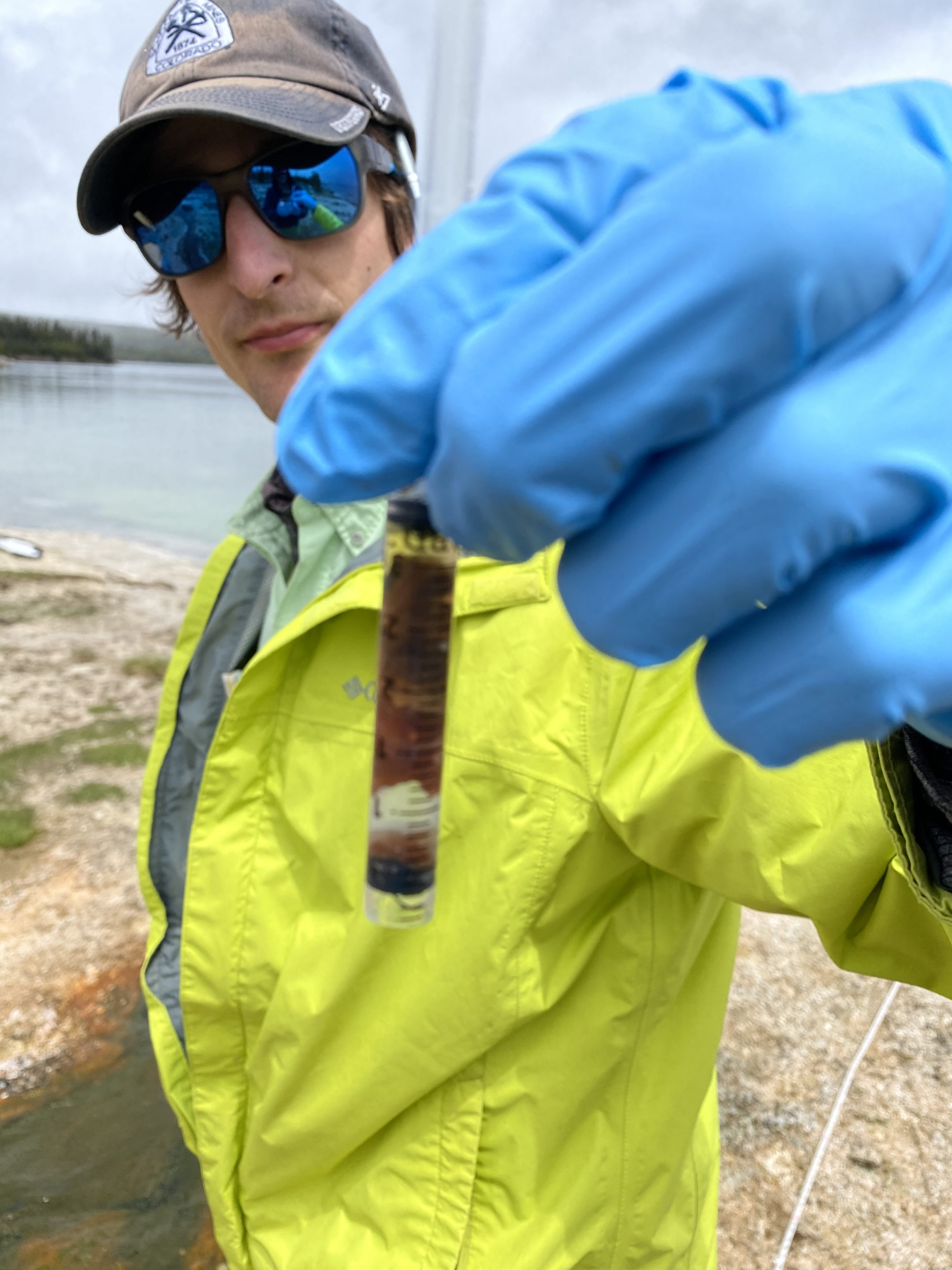
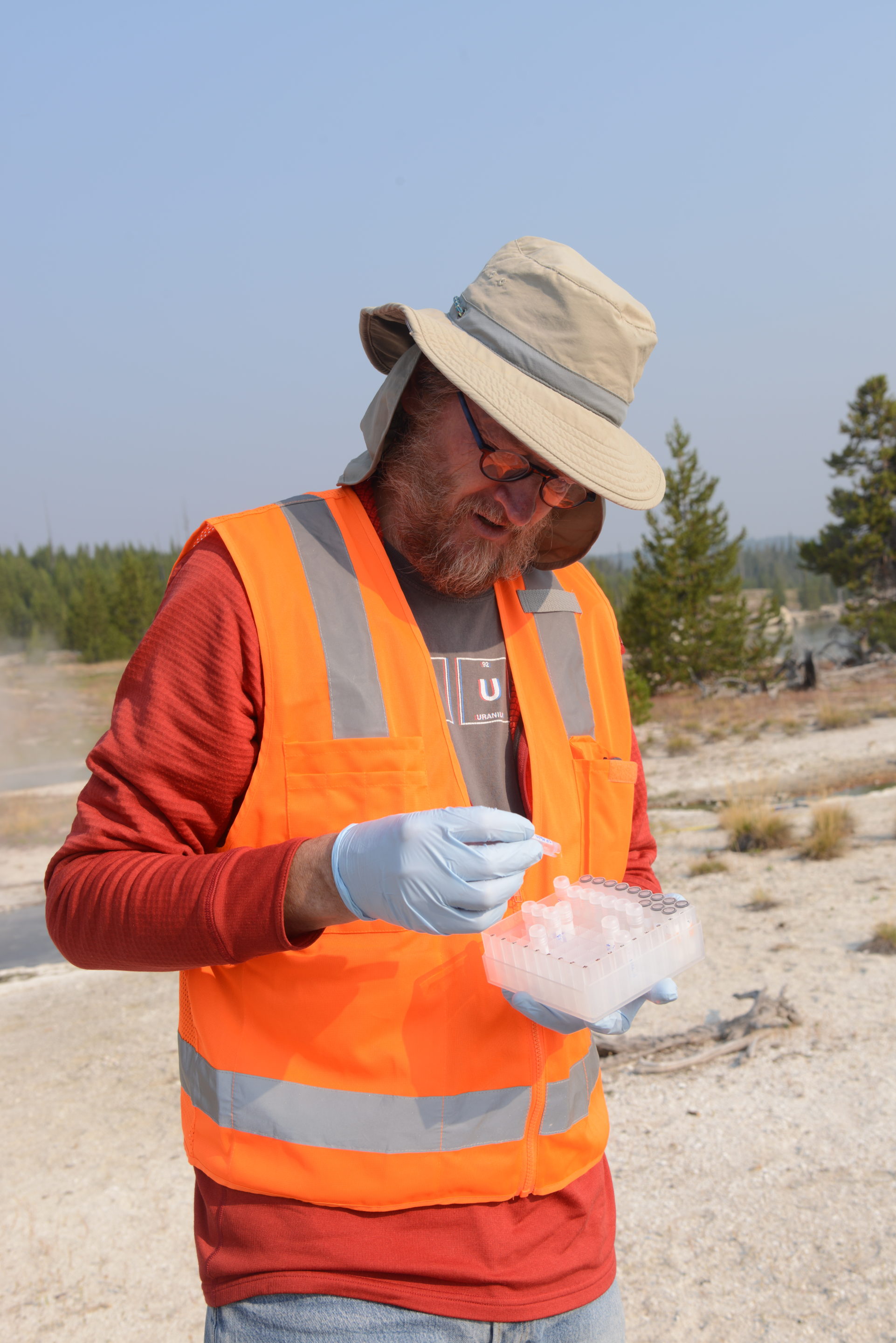
About: What We Do
Currently, members of this research group are engaged in a number of activities. The link between these activities is something microbial and the methodology by which we study the particular problem. Traditional microbiology has taught us much about the diversity of the microbial world. Particularly with regard to what microbiota look like and their biochemical processes. While it remains quite true, “That to know it-you have to grow it,” we now know that the bulk of the microorganisms in the environment are incapable of being brought into cultivation. It is too difficult to duplicate an organism’s host environment/ecosystem in a dish in a laboratory.
With the advent of modern molecular techniques, we can understand the microbial environment in much greater detail through an examination of DNA present in any environment. Phylogenetic characterization is possible by base-pair comparison of DNA sequence between organisms. Applied to genetically conserved genes, like small sub-unit ribosomal rRNA genes (SSU rRNA), we can begin to understand how organisms are related. We can also look at functional genes-genes that help to facilitate specific pathways like nitrogen fixation, metal reduction or hydrogen utilization. With Next-Generation sequencing approaches we can also now ask whole single-cell genomics questions of any environment. The comparisons of multiple genomes form a single environment, or between multiple environments, will no doubt better unlock what is known of the microbial world.
Typically our studies take us to a field site where some microbial process is occurring or is thought to. We sample and characterize the site for associated chemistry/geochemistry, and collect material for DNA analysis back in the laboratory. At the bench, we employ molecular processes of DNA extraction, polymerase chain reaction (PCR), quantitative PCR (qPCR), DNA sequencing (currently via Illumina DNA sequencing platforms), phylogenetic tree building, single-cell genomic comparisons and bioinformatic interpretation with known and novel tools to statistically compare microbial communities.
Our laboratory is stocked with state of the art equipment that includes, anaerobic chambers, PCR machines, spectrophotometers, “sterile” work spaces, a Leica analytical microscope, an Hitachi TM-1000 Scanning Electron Microscope (SEM) and several computer workstations for data manipulation.
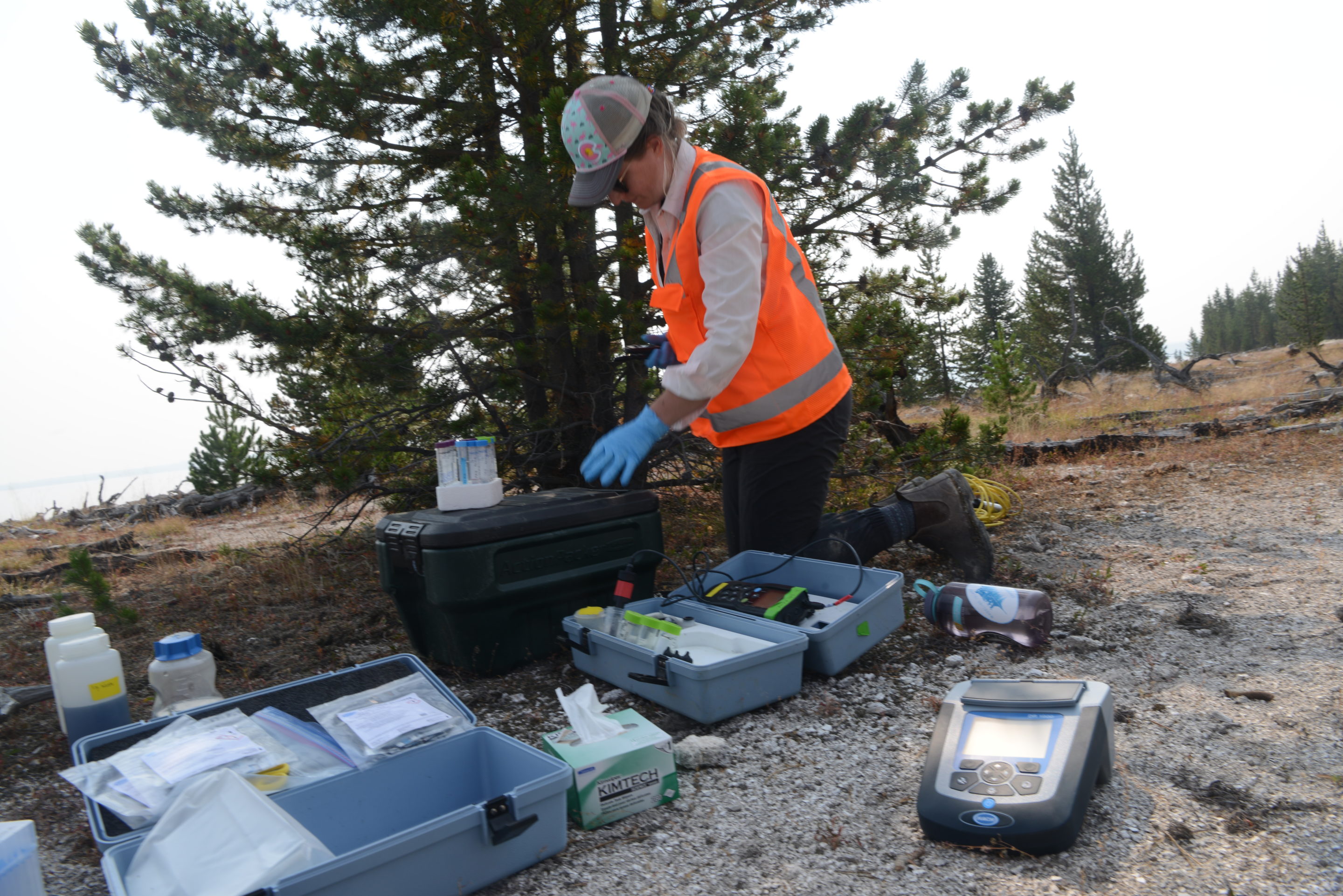
research interests
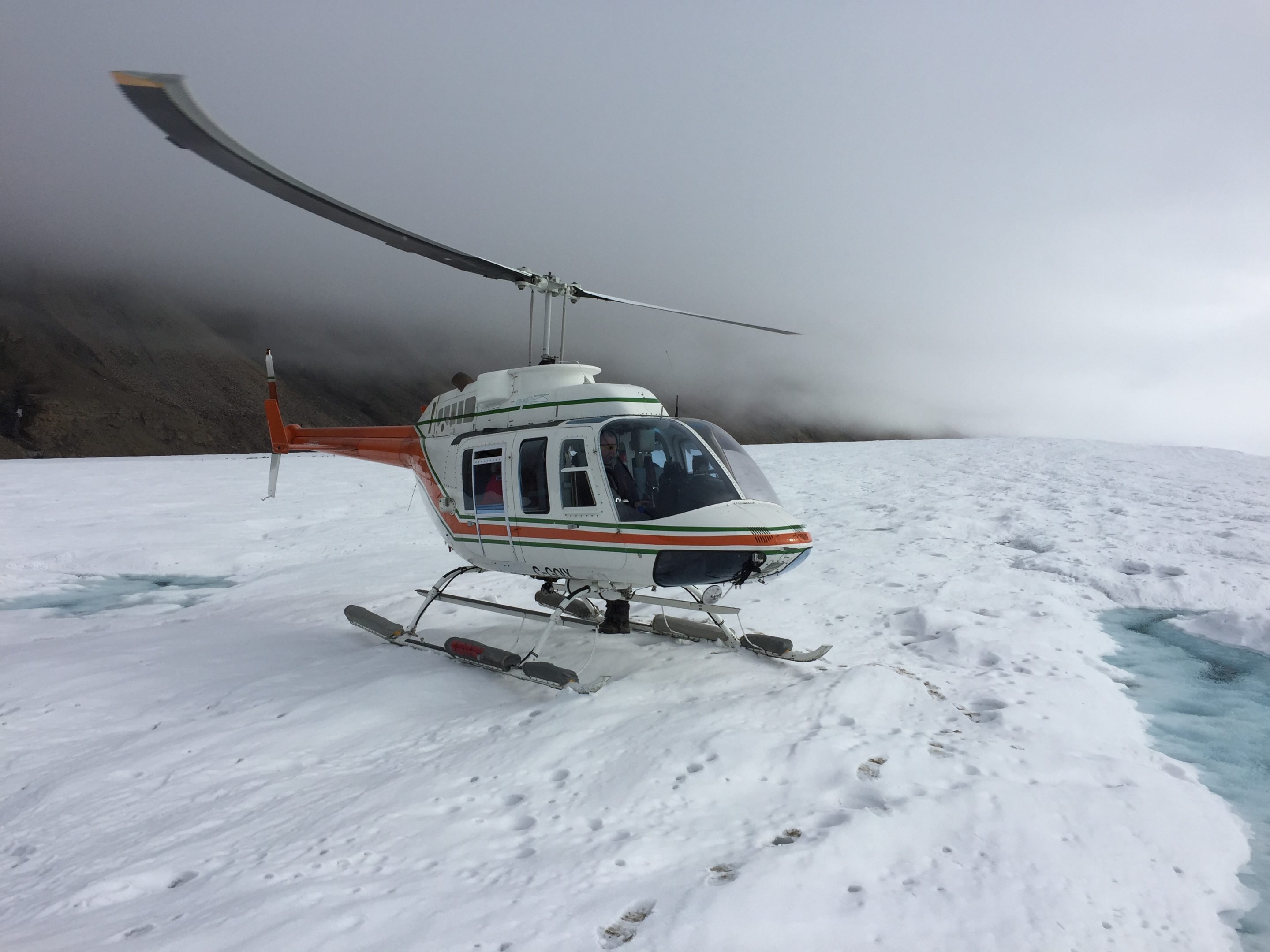
ASTROBIOLOGY AND ELEMENTAL SULFUR DEPOSITS AT BORUP FIORD PASS GLACIER
Microbial activity can control the abundance and distribution of sulfur species in environments far from chemical equilibrium, including the precipitation of intracellular and extracellular elemental sulfur in association with organic structures such as surface coatings, cell surfaces, filaments and sheaths. However, the potential for generation and preservation of S0 mineral deposits that could serve as biosignatures has not been extensively investigated. The objective of this proposal is to take advantage of the annually recurring large-scale formation of an icy elemental sulfur deposit at Borup Fiord Pass in the Canadian High Arctic and to define direct feedbacks between the aqueous geochemistry, microbial community composition, genetic activity and the chemical speciation of S0-rich deposits during their rapid accumulation.
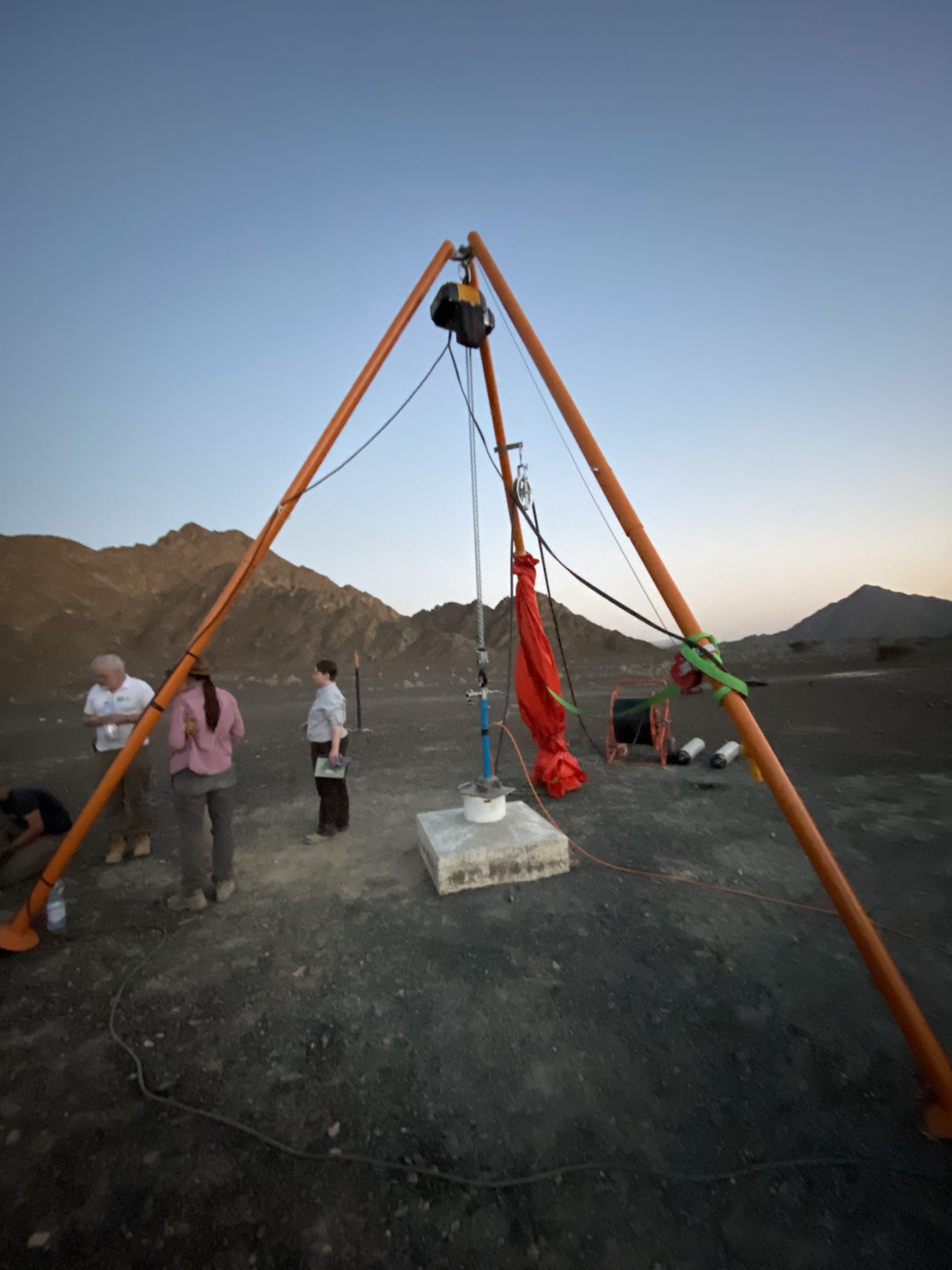
MICROBIAL INFLUENCED CORROSION PROJECT
Microbially influenced corrosion (MIC) is a huge problem to any metallic infrastructure, anywhere. As such, the “decay” of vital pipelines, steel tanks, ships and other delivery mechanisms costs the U.S. billions of dollars per year to replace. In a grant funded by the U.S. Department of Transportation Pipeline and Hazardous Materials Safety Administration, we are trying to assess the impact of microbes on various strengths of steel that come into contact with ethanol and ethanol-containing products (e.g. E85, ethanol: gasoline mixes). With the rapid growth of ethanol production and consumption as an alternative and additive to liquid fuels, the conveyance of this important fluid is important to lower costs. For example, most ethanol produced in the US today is transported by truck to avoid damage to the existing pipe and tank infrastructure for fear of corrosion by two mechanisms–both stress corrosion cracking (SCC) and MIC. At the heart of this project, we are determining what kinds of microbiota produce the most damage and the mechanisms by which they do so.
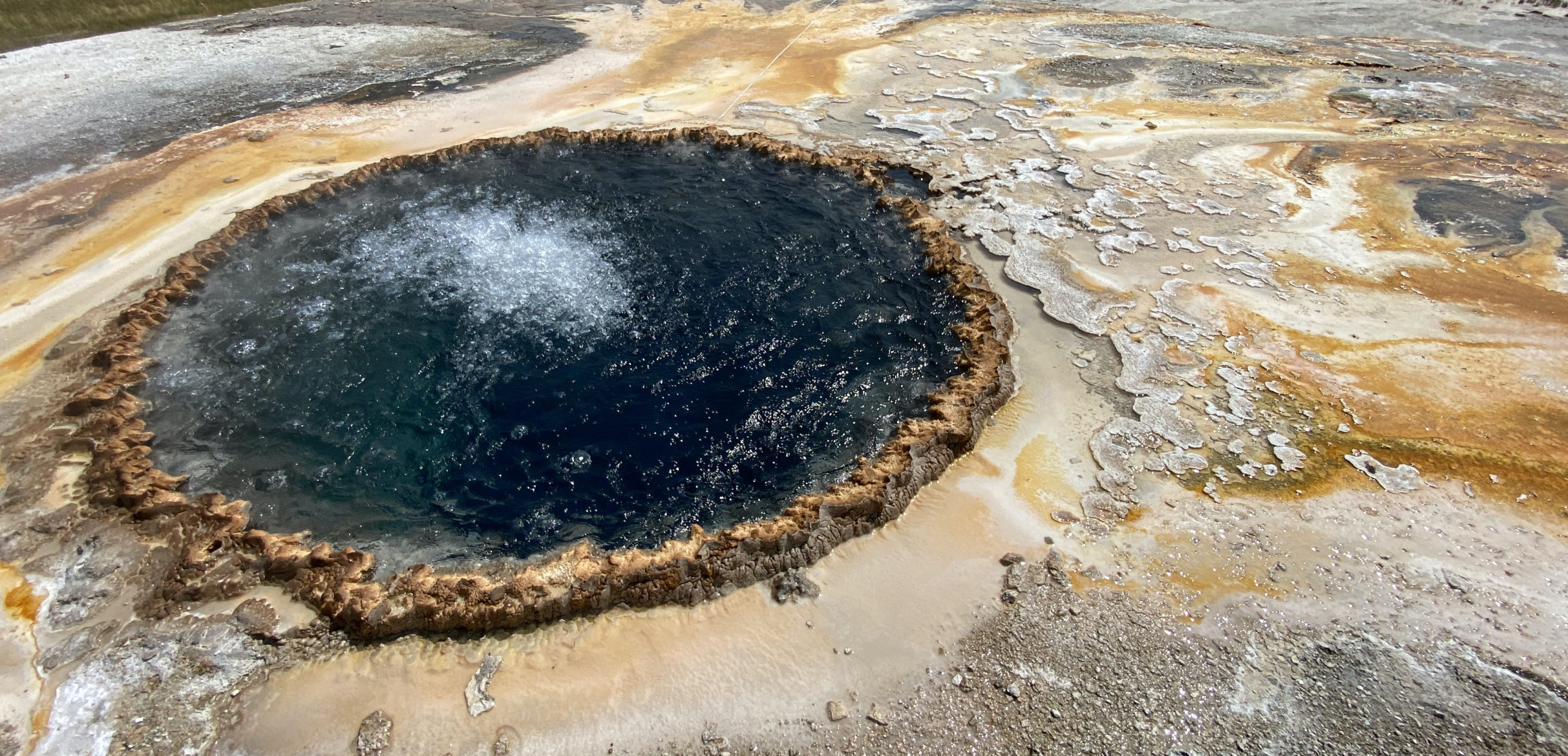
ENVIRONMENTAL HYDROGENASES IN GEOTHERMAL HOTSPRINGS
This is an NSF funded project that extends work done as a Postdoc in Norm Pace’s laboratory. We have shown that many, if not most, microbial communities in Yellowstone National Park hotsprings rely upon aqueous molecular hydrogen (H2) concentrations (nM) as a source of electrons to carry out metabolism. Now, we seek to show how this process happens. Presumably, microbial cells of many different kinds, have functional hydrogenase enzymes oxidizing the hydrogen to water. Most hydrogenase enzymes will also carry out this reaction in reverse, producing hydrogen. Thus, this work has functional components to both basic science, i.e., what happens in nature; and the potential for engineered application in the area of bioenergy, i.e., use of microbially produced hydrogen as a fuel source.
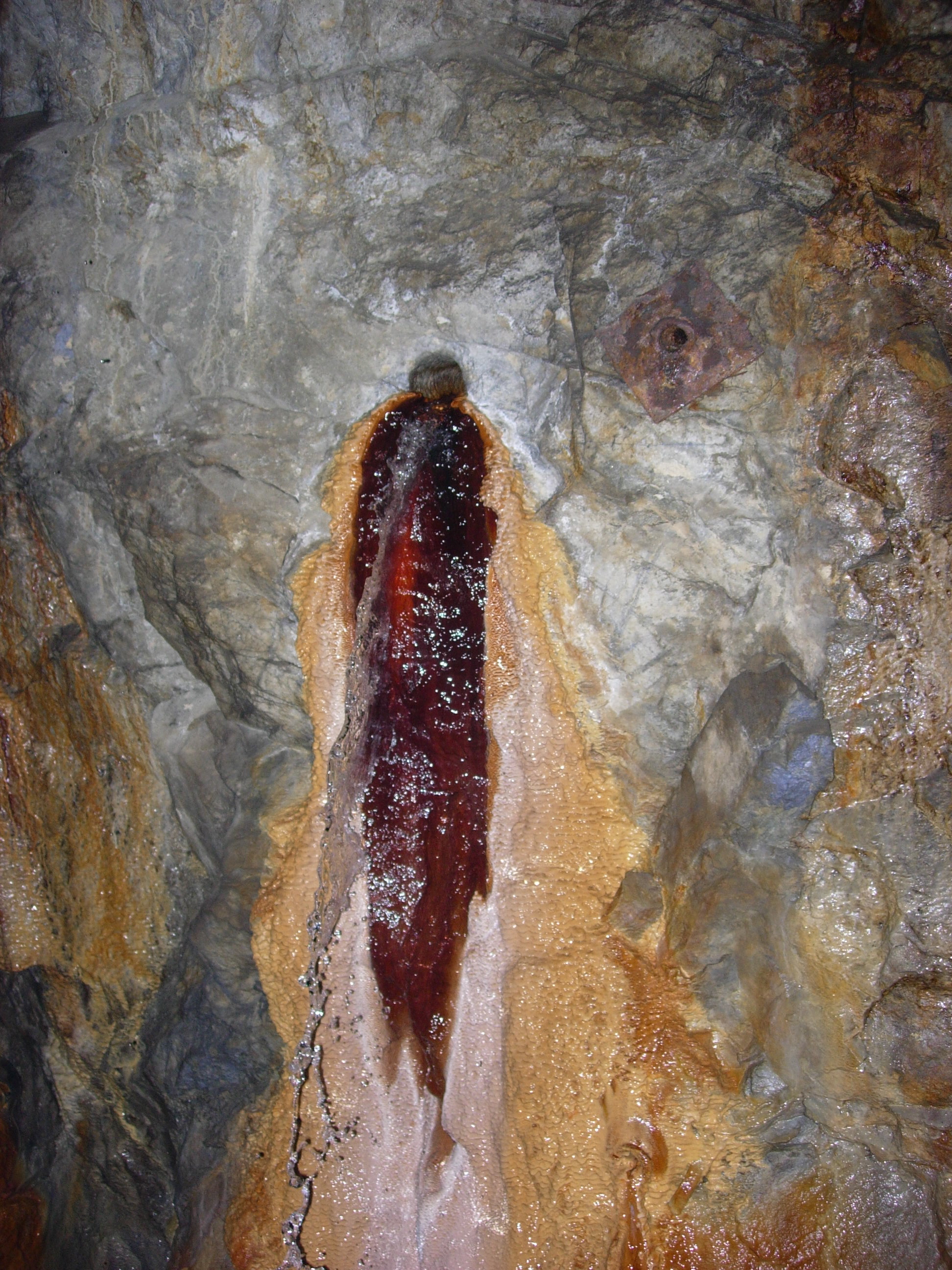
MICROBES AND CAVES PROJECT
Microbiota that live in caves provide a huge link between the geochemistry of the cave system, the formation of the cave (speleogenesis) and the minerals and formations (speleothems like stalactites) that lie within them. In addition to these important attributes, caves are an accessible place to better understand subsurface microbiology and geochemistry. We have been working on various cave-related projects for a number of years. One of our projects showed how microbiota may lie at the heart, and are instrumental for, the formation of ceiling-bound stalactites; and that nitrogen cycling in a cave system may be actively done by Ammonia-Oxidzing Archaea (AOA). Other projects that we are working on, without funding, are sulfur-based cave ecosystems in both Colorado and Wyoming. These ecosystems are unique and valuable for the better understanding of the S cycle in any environment. To better understand the subsurface world is to better understand the surface world–where most of us are, most of the time!
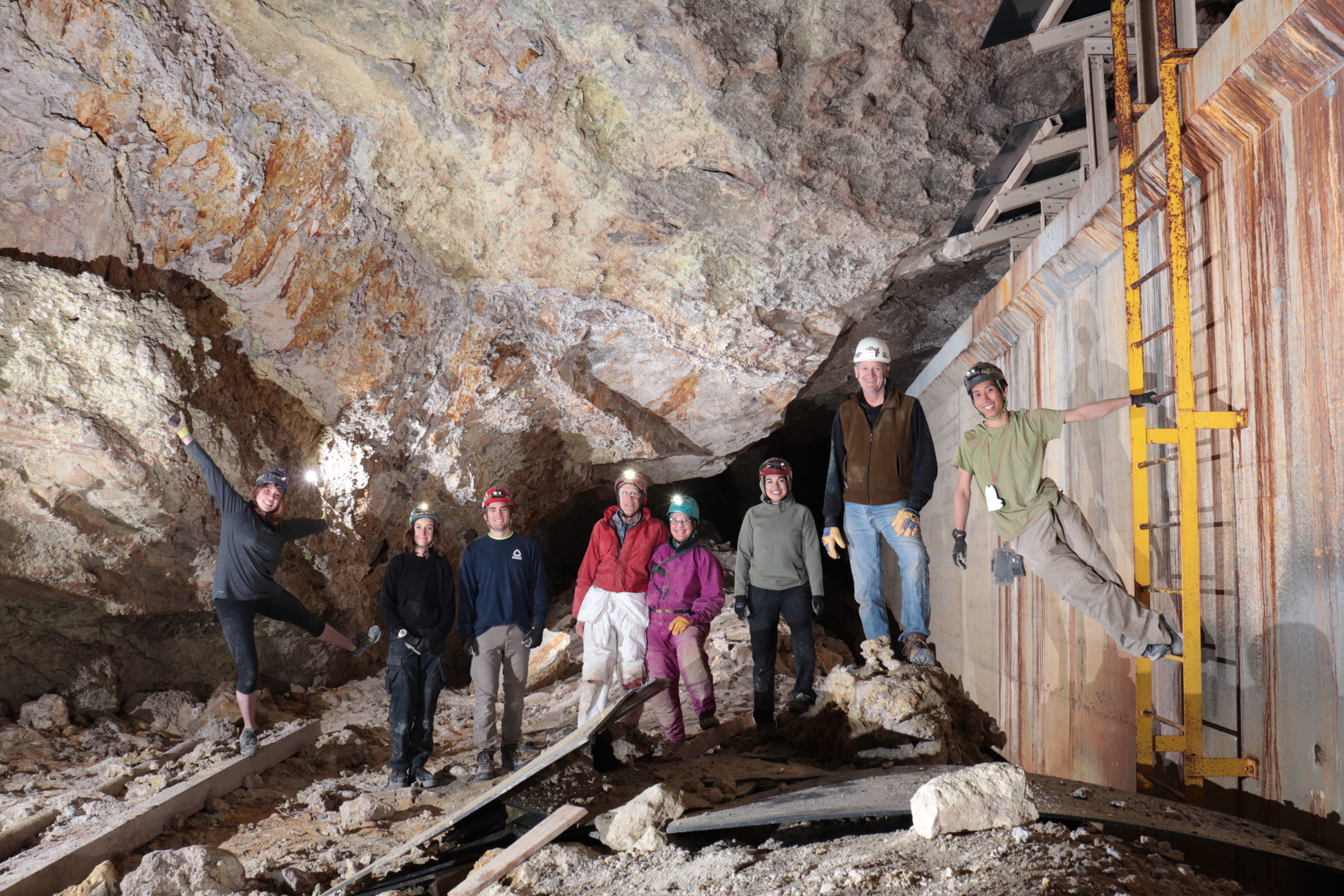
GEOBIOLOGY INTERESTS
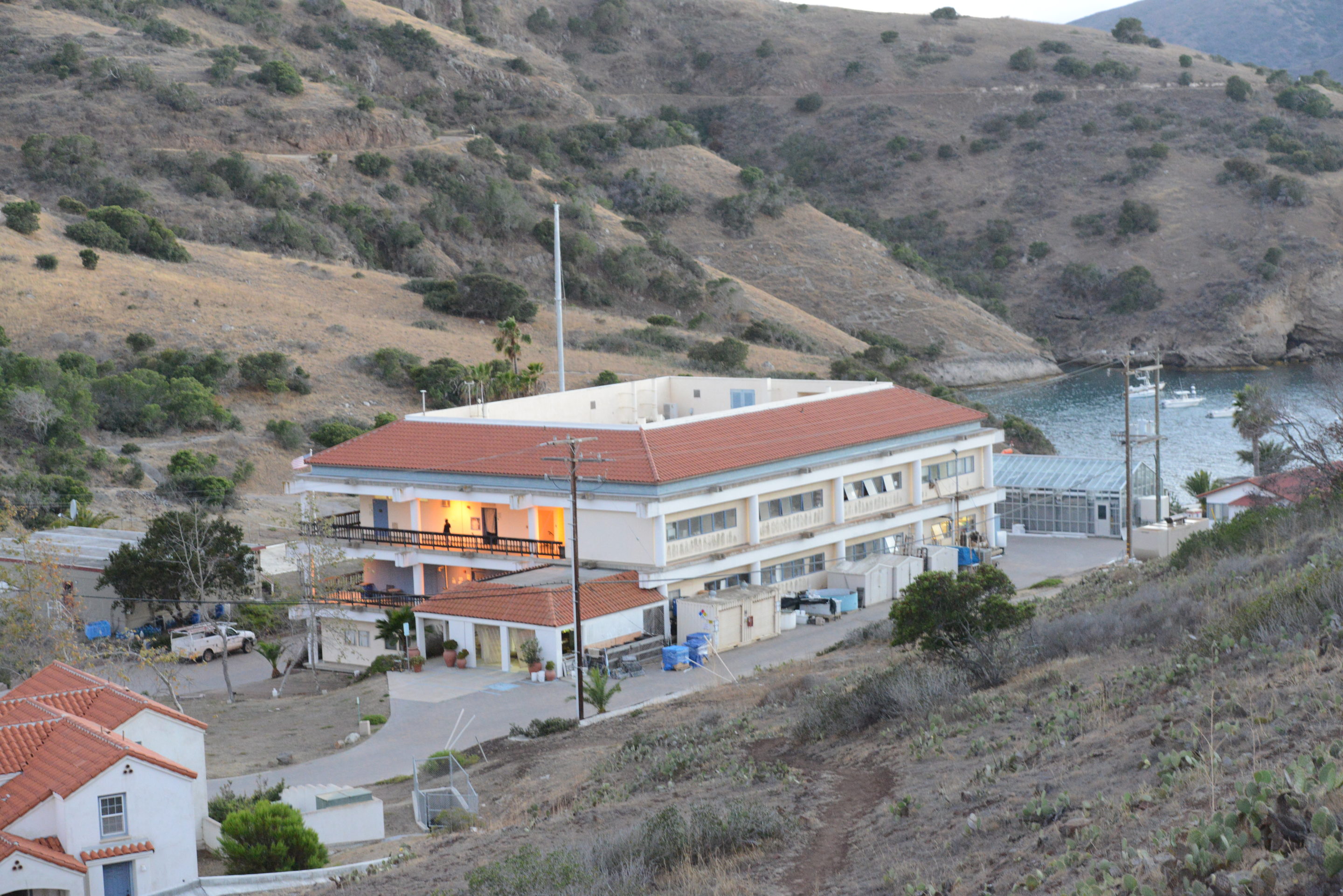
For several years I have been thinking about the rock: microbiota interface. How ore bodies form and what microbial processes are involved, for example. For my Ph.D. work, I looked at the kinetics of how fast sulfate-reducing bacteria can reduce soluble U(VI) to insoluble U(IV). This process could have been responsible for the formation of natural uranium ore bodies, but can now be examined and then utilized for the removal of U in contaminated waters for remediation purposes. I then moved on to characterize the microbial communities present in the “endolith”-microbes located in the pore spaces of rocks, with a focus on gypsum/halite evaporite salts. Currently, we are looking at the communities that make up the subsurface ecosystem of the Samail Ophiolite, Sultanate of Oman, a serpentinized system that appears to exhibit a rock-hosted microbiome and a fluid-hosted microbiome–and they are different. The rock/microbe interface is quite amazing in any environment; from the sidewalk under your feet to the crag you climb on to the deep subsurface!
From 2010-2016, I co-directed, along with Frank Corsetti from the University of Southern California, the International GeoBiology Course: an intense summer training course for graduate students to obtain advanced, state-of-the-art knowledge on the subject and field of GeoBiology (dornsife.usc.edu/wrigley/geobiology/). The course set-up varies from year to year but typically involves field time, lab time, data analysis, and group projects. This course is very unique, very intense, and insures a place where much can be learned!
previous projects
The Fish Food Project
The Fish Food Project
In a grant to the National Science Foundation’s Materials Use: Science, Engineering and Society (MUSES) program entitled “Production and use of a novel bacterial protein in aquaculture operations: societal benefits and global ramifications,” we sought to take what has normally been considered a waste stream-bacterial biomass from food production-and turn that into a beneficial source of protein for farm-raised fish. To do this, we needed to characterize the kinds of microbiota present in single-cell protein biomass obtained from a brewery waste stream. With that information, we can design and construct machinery to make a high protein content additive for fish meal. Farm-raised tilapia can then eat the high protein meal, and farmers can get bigger fish, ready faster, for the marketplace. Some aspects of this work have both anthropogenic and economic ramifications, and these were explored by partners at the University of Colorado, Boulder, and Yale University.
For further information, check the website of our corporate collaborators, Nutrinsic, Inc. at www.gonutrinsic.com.
The DEep Phreatic THermal eXplorer (DEPTHX)
The DEep Phreatic THermal eXplorer (DEPTHX)
Funded by a NASA Astrobiology Science and Technology for Exploring Planets (ASTEP) grant, this project sought to develop an autonomous underwater vehicle (AUV) that is capable of mapping, exploring, and sampling its world in 3-D space. The team responsible for this idea and work includes people from CSM, Carnegie-Mellon University, The University of Texas, and the Southwest Research Institute. The robot was built by the lead design team members at Stone Aerospace in Austin, TX, and was deployed in Cenoté Zacatón, ~2 hours NW of Tampico, Mexico, in the Spring of 2007. This exciting project had as a goal, the sampling of microbial life from deep within the Cenoté, returning that sample to the surface, and telling us where it came from. A variety of instruments and algorithms were on board to allow a choice of sampling place. Samples collected were both water column samples and biofilm/wall samples that were delivered back to our laboratory for molecular phylogenetic characterization.
Some useful websites for more information are:
- https://www.astrobio.net/news-exclusive/robot-dives-deep-for-sinkhole-slime/
- https://www.astrobio.net/robotics-a-i/demystifying-el-zacata³n/
- https://www.astrobio.net/extreme-life/diving-robot-discovers-life-in-the-slow-lane/
- https://spectrum.ieee.org/computing/hardware/swimming-to-europa
- A blog of our activity can be found here: http://www.stoneaerospace.com/news-/news-zacaton-mission1.php